Medical Radionuclide Production
Common Radionuclides In Medicine
Brachytherapy Sources
Nuclide | Half-life | Photon Energy (MeV) | HVL (mm Lead) | Exposure Rate Constant (R-cm2/mCi-hr) |
---|---|---|---|---|
192Ir | 73.8 days | 0.38 average | 2.5 | 4.69 |
226Ra | 1,600 years | 0.83 average | 12.0 | 8.25 |
222Rn | 3.83 days | 0.83 average | 12.0 | 10.15 |
60Co | 5.26 years | 1.17, 1.33 | 11.0 | 13.07 |
137Cs | 30.0 years | 0.662 | 5.5 | 3.26 |
198Au | 2.7 days | 0.412 | 2.5 | 2.38 |
125I | 59.4 days | 0.028 average | 0.025 | 1.46 |
103Pd | 17.0 days | 0.021 average | 0.008 | 1.48 |
PET Nuclides
Nuclide | Half-life (minutes) | Positron Maximum Energy (MeV) | Dose rate constant (μSv m2/MBq) |
---|---|---|---|
18F | 109.8 | 0.63 | 0.143 |
11C | 20.4 | 0.96 | 0.148 |
13N | 10.0 | 1.19 | 0.148 |
15O | 2.0 | 1.72 | 0.148 |
64Cu | 762 | 0.65 | 0.029 |
68Ga | 68.3 | 1.9 | 0.134 |
82Rb | 1.27 | 3.35 | 0.159 |
124I | 6048 | 1.54, 2.17 | 0.185 |
A Closer Look At Medical Nuclides
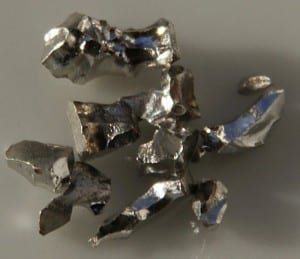
Ir-192
Iridium-192 (192Ir) is the most common isotope used in high dose rate (HDR) brachytherapy. It is a man-made isotope produced by neutron bombardment of Iridium-191. (192Ir) decays primarily through β- decay to an excited state of Platinum-192 which is stable. Approximately 5% of decays occur via electron capture yielding an excited state of Osmium-192 which is also stable. It is regarded as superior to cobalt-60 and cesium-137 due to its higher specific activity which allows for smaller source sizes. One disadvantage to (192Ir) is its short half life (73.83 days) which results in a loss of activity of approximately 1% per day. This necessitates source changes every 3-4 months.
- Half-life: 73.83 days
- Activity loss per day (quick estimate): 1%/day
- Decay modes
- 95.6% β- decay to excited state of 192Pt
- 4.4% Electron capture to excited states of 192Os
- Exposure rate constant
- Air kerma rate constant
- Photon energy
- 0.136-1.06 (0.38 average) MeV
- Half-value layer (mm lead): 2.5mm
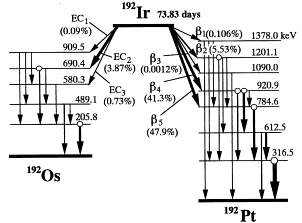
IR-192 decay scheme
Methods of Radionuclide Production
Radionuclides used in nuclear medicine have a short half-life which allows for faster treatments, faster imaging, and lower public exposure. As a result of the need for such short half-lives, most radiopharmaceuticals in use today must use artificially generated radionuclides. The vast majority of these nuclides are produced in cyclotrons, nuclear reactors, and radionuclide generators.
Nuclear Transformation Notation
Nuclear transformations are denoted T(P, E)R where T is the target material, P is the accelerated particle type, E is the number and type of emitted particles during transformation, and R is the resulting radionuclide.
For example, the notation for Gallium-67 production is 68Zn (p, 2n) 67Ga which indicates that a proton (p) was accelerated into 68Zn which produced 67Ga by the emission of two neutrons (2n).
Often, the desired radionuclide is produced after decay of a bombardment produced radionuclide.
For example, Iodine-123 is produced via the (p, 5n) transformation to Xe-123 which then undergoes electron capture (EC) to yield I-123.

Nuclear fission resultant of neutron absorption is indicated as in the below example. The creation of Mo-99 (a parent of Tc-99m) via thermal neutron bombardment and subsequent decay of U-235. Note that in addition to Mo-99 and Sn-134, three neutrons and additional gamma photons are produced with a net energy release of about 200 MeV.

Cyclotrons
A cyclotron is a charged particle accelerator consisting of an evacuated cylinder divided into two sections referred to as "Dees" (because they look like a capital D).The evacuated cylinder is placed between two large magnets producing a constant magnetic field. Charged particles are injected into the center of the cylinder and an oscillating electric field is applied to the Dees. The electric field causes the charged particles to accelerate and the constant magnetic field causes that acceleration to have a circular trajectory. The energy and velocity of the particles increase as they pass from one Dee to the other.
Radionuclides are produced by colliding these high energy-charged particles into target nuclei. The accelerated particle type, its energy, and the target material all impact what nuclides will be produced. Cyclotron-produced nuclei will be created in an excited state and will decay either by particle (protons and neutrons) or electromagnetic (photon) emissions. Most cyclotron-produced radionuclides are neutron deficient and decay via either electron capture (EC) or positron emission (β+).
Key Point: Most Positron Emission Tomography (PET) radionuclides are produced in cyclotrons.
Nuclear Reactors
Nuclear reactors are commonly used to produce radionuclides via nuclear fission or nuclear activation.
Nuclear Fission
Nuclear fission is the splitting of a heavy atom into multiple smaller nuclei. Nuclear fission is induced in a nuclear reactor by the absorption of thermal (low energy) neutrons. Medical radionuclides may be produced as a decay product of these nuclear fusions.
Nuclear Activation
Neutrons produced in a nuclear reactor may also be used to bombard stable nuclei in a process known as nuclear activation. When the stable nucleus absorbs a neutron, it produces an isotope with a moderate half-life.
The most common neutron activation methods are the (n, γ) reaction, in which neutron capture is immediately followed by emission of a gamma photon, and the (n, p) or (n, α) reactions in which a proton or alpha particle is emitted immediately after neutron absorption.
Radionuclide Generators
Radionuclide generators produce a short-lived medical radionuclide by decay of a longer-lived radionuclide. The most common example of a clinically useful radionuclide generator is the Molybdenum-99/Technitium-99m generator often referred to as Moly Cows.
Moly Cows (Tc-99m Generators)
In a Moly Generator, a sample of Mo-99 (in the form of ammonium molybdenate) is loaded into a porous column containing aluminum oxide resin. The ammonium molybdenite becomes attached to the surface of the resin, greatly increasing its surface area. When the Mo-99 decays to Tc-99m, the Tc-99m is less tightly bound to the resin than its parent, Mo-99. This allows the Tc-99m to be washed from the resin and collected while leaving the Mo-99 behind. This washing process is referred to as “milking” and gives rise to the name Moly Cow (i.e. “milking the cow”).
Navigation
Not a Premium Member?
Sign up today to get access to hundreds of ABR style practice questions.